Additive manufacturing, commonly known as 3D printing, has emerged as a transformative technology revolutionizing traditional manufacturing processes across industries. Its ability to fabricate intricate designs, customize products, and streamline production has garnered widespread attention and adoption. In this comprehensive guide, we delve into the world of additive manufacturing, exploring its definition, various types, real world examples, and detailed explanations. From aerospace to healthcare, automotive to consumer goods, additive manufacturing has reshaped the landscape of product development and manufacturing. Join us as we unravel the intricacies of additive manufacturing, uncovering its advantages, applications, and the future it promises to shape.
What Is Additive Manufacturing?
Additive manufacturing, often referred to as 3D printing, is a process of creating three dimensional objects by adding successive layers of material one upon the other. Unlike traditional subtractive manufacturing methods where material is cut away from a solid block, additive manufacturing builds up the object layer by layer from digital 3D models. This process allows for highly intricate and customized designs to be produced with relative ease, making it popular in various industries such as aerospace, automotive, healthcare, and consumer goods. Additive manufacturing offers advantages such as rapid prototyping, reduced material waste, and the ability to create complex geometries that may be difficult or impossible to achieve through traditional manufacturing methods.
Additive Manufacturing Definition:
Additive manufacturing is a manufacturing process that builds objects layer by layer using materials like plastics, metals, ceramics, or composites, based on digital 3D models. This method contrasts with traditional subtractive manufacturing, where material is cut away from a solid block. Additive manufacturing enables the creation of complex shapes and structures with high precision and customization, making it suitable for various industries including aerospace, automotive, healthcare, and consumer goods.
Types of Additive Manufacturing:
Additive manufacturing, also known as 3D printing, encompasses a variety of techniques for creating three dimensional objects by adding material layer by layer. Each type of additive manufacturing process has its own unique advantages, applications, and considerations. Below, we explore some of the most common types of additive manufacturing:
1. Material Extrusion
Material extrusion, also known as fused deposition modeling (FDM) or fused filament fabrication (FFF), is one of the most widely used additive manufacturing techniques. In this process, thermoplastic filaments are heated and extruded through a nozzle, forming successive layers to build up the desired object. FDM is known for its versatility, affordability, and accessibility, making it popular for rapid prototyping, hobbyist projects, and educational purposes.
2. Stereolithography (SLA)
Stereolithography (SLA) utilizes a liquid photopolymer resin that is cured (solidified) layer by layer using a UV laser or other light sources. SLA produces highly detailed and accurate parts with smooth surface finishes, making it ideal for applications requiring precision and intricate geometries. SLA is commonly used in industries such as jewelry, dentistry, and prototyping of high-resolution parts.
3. Selective Laser Sintering (SLS)
Selective laser sintering (SLS) involves using a high-powered laser to selectively fuse powdered materials, such as plastics, metals, or ceramics, layer by layer to create the final object. SLS offers the advantage of producing functional parts with good mechanical properties and a wide range of material options. It is commonly used in aerospace, automotive, and manufacturing industries for producing end-use parts and functional prototypes.
4. Direct Metal Laser Sintering (DMLS)
Direct metal laser sintering (DMLS) is a variation of SLS specifically designed for metal materials. In DMLS, metal powder is selectively fused together using a high powered laser to create metal parts with high density and precision. DMLS is widely used in aerospace, medical, and automotive industries for producing complex metal components with excellent mechanical properties.
5. Electron Beam Melting (EBM)
Electron beam melting (EBM) is another metal additive manufacturing process that uses an electron beam to melt and fuse metal powder together layer by layer. EBM offers advantages such as faster build times and the ability to produce parts with minimal residual stress. It is commonly used in aerospace, healthcare, and energy sectors for producing high-performance metal parts, including implants, turbine blades, and aerospace components.
6. Binder Jetting
Binder jetting is an additive manufacturing process where a liquid binding agent is selectively deposited onto a powder bed, binding the particles together to form each layer of the object. Binder jetting offers high speed and cost effectiveness, especially for producing large parts or prototypes. It is commonly used in industries such as foundry, automotive, and aerospace for producing sand molds, investment casting patterns, and metal prototypes.
7. Material Jetting
Material jetting is an additive manufacturing process that operates similarly to inkjet printing. It involves jetting droplets of liquid photopolymer onto a build platform, which are then cured (solidified) layer by layer using UV light. Material jetting offers high resolution and the ability to produce multi material and full color parts, making it suitable for applications such as product design, prototyping, and architectural modeling. While material jetting is known for its exceptional surface finish and detail, it may have limitations in terms of material properties and production speed.
8. Powder Bed Fusion (PBF)
Powder bed fusion (PBF) encompasses several additive manufacturing processes, including selective laser melting (SLM) and electron beam melting (EBM), where metal or plastic powders are selectively fused together using a high energy source. PBF techniques offer high precision and the ability to produce fully dense metal parts with complex geometries. These processes are widely used in aerospace, medical, and automotive industries for producing lightweight, high-performance components such as turbine blades, implants, and custom tooling.
9. Continuous Liquid Interface Production (CLIP)
Continuous Liquid Interface Production (CLIP) is an innovative additive manufacturing process developed by Carbon Inc. It utilizes a combination of light and oxygen to rapidly cure a liquid resin into a solid part. CLIP offers high-speed production, excellent surface finish, and isotropic mechanical properties, making it suitable for producing end-use parts with engineering grade materials. CLIP has gained traction in industries such as automotive, consumer electronics, and medical devices for producing functional prototypes, production parts, and custom products.
10. Hybrid Additive Manufacturing
Hybrid additive manufacturing combines additive manufacturing processes with traditional subtractive machining operations in a single machine setup. This approach allows for the production of parts with complex geometries that may require both additive and subtractive methods. Hybrid systems offer benefits such as improved surface finish, reduced material waste, and enhanced part quality. They are increasingly being adopted in industries such as aerospace, mold and die, and tooling for producing high-performance components with optimized designs and reduced lead times.
Future Directions
As additive manufacturing technology continues to advance, researchers and industry experts are exploring new materials, processes, and applications to further expand its capabilities. Future developments may include advancements in multi material printing, improved process monitoring and control, integration of artificial intelligence and machine learning, and the adoption of sustainable materials and processes. With ongoing innovation and collaboration across disciplines, additive manufacturing is poised to revolutionize manufacturing processes, supply chains, and product designs in the years to come.
Metal Additive Manufacturing:
Metal additive manufacturing, also known as metal 3D printing, is a cutting edge manufacturing process that builds metal parts layer by layer from digital 3D models. This technology allows for the creation of highly intricate and complex metal components that may be difficult or impossible to produce using traditional manufacturing methods.
In metal additive manufacturing, various techniques are employed, including:
Powder Bed Fusion:
This technique involves spreading a layer of metal powder over a build platform and then selectively melting or sintering the powder using a laser or electron beam according to the digital design.
Directed Energy Deposition:
In this method, a focused energy source, such as a laser or electron beam, is used to melt metal powder or wire as it is deposited onto a substrate, allowing for the fabrication of large, near net shape parts or the repair and modification of existing components.
Binder Jetting:
Metal powder is selectively deposited onto a build platform in thin layers, with a liquid binding agent applied to bind the particles together. After each layer, the build platform is lowered, and a new layer of powder is spread, repeating the process until the object is complete.
Metal additive manufacturing offers several advantages, including the ability to produce complex geometries with high precision, reduced material waste, faster prototyping, and the potential for lightweight designs. It is widely used in industries such as aerospace, automotive, healthcare, and tooling for applications ranging from rapid prototyping to the production of end-use parts. However, challenges such as material properties, surface finish, and post-processing requirements need to be addressed for certain applications.
3d printing and Additive Manufacturing:
3D printing and additive manufacturing are often used interchangeably, but there are subtle differences between the two terms.
Additive manufacturing (AM) is the broader term that encompasses various techniques for creating three dimensional objects by adding material layer by layer based on digital 3D models. It includes not only the popular desktop 3D printing technologies but also industrial grade processes used in manufacturing.
3D printing specifically refers to the process of creating physical objects from digital models using a 3D printer. While 3D printing is a form of additive manufacturing, not all additive manufacturing processes are necessarily 3D printing. 3D printing is typically associated with desktop or small scale printers that use materials such as plastics, resins, or filaments to create objects for prototyping, hobbyist projects, and small scale production.
In summary, additive manufacturing is the overarching term that encompasses all processes that build objects layer by layer from digital models, while 3D printing specifically refers to the subset of additive manufacturing processes that use 3D printers to create objects, often on a smaller scale and with materials like plastics or resins.
Progress In Additive Manufacturing:
Progress in additive manufacturing has been remarkable in recent years, with advancements occurring in various aspects of the technology, including materials, processes, applications, and scalability. Here are some key areas of progress:
1. Materials Development:
Additive manufacturing has witnessed significant progress in materials development, with the introduction of new polymers, metals, ceramics, and composites optimized for specific additive manufacturing processes. These materials offer improved mechanical properties, thermal stability, and chemical resistance, expanding the range of applications for additive manufacturing in industries such as aerospace, automotive, healthcare, and consumer goods.
2. Process Innovation:
There have been continuous innovations in additive manufacturing processes aimed at enhancing speed, precision, and scalability. Techniques such as binder jetting, directed energy deposition, and vat photopolymerization have been refined to achieve higher resolution, faster build times, and reduced post-processing requirements. Process optimization efforts have also focused on improving surface finish, dimensional accuracy, and material utilization efficiency.
3. Scalability and Production Integration:
Additive manufacturing technologies have evolved to accommodate larger build volumes and higher throughput rates, enabling their integration into production environments for serial manufacturing of end-use parts. Advancements in automation, robotics, and software control have streamlined workflow processes, minimized operator intervention, and improved overall manufacturing efficiency. These developments have paved the way for additive manufacturing to be adopted for mass customization, on-demand manufacturing, and distributed production networks.
4. Multi-Material and Functionally Graded Structures:
Progress in additive manufacturing has enabled the fabrication of multi material and functionally graded structures with tailored mechanical, thermal, and electrical properties. By combining different materials within a single part or layer-by-layer, additive manufacturing techniques can create complex geometries and material compositions that are not achievable with traditional manufacturing methods. This capability has opened up opportunities for designing lightweight components, heat exchangers, and medical implants with optimized performance characteristics.
5. Design Optimization and Generative Design:
Additive manufacturing has facilitated the implementation of generative design algorithms and topology optimization techniques to create organic, lattice like structures that maximize strength to weight ratios and material utilization. By leveraging the design freedom afforded by additive manufacturing, engineers can create optimized geometries that minimize material usage while maintaining structural integrity and functional performance. This approach has revolutionized product design and has led to the development of innovative components in various industries.
6. Sustainability and Circular Economy:
Progress in additive manufacturing has also contributed to sustainability initiatives and the circular economy by reducing material waste, energy consumption, and carbon emissions compared to traditional manufacturing methods. Additive manufacturing enables on-demand production, localized manufacturing, and design for recyclability, facilitating the transition towards more sustainable and environmentally friendly manufacturing practices. Additionally, bio-based and recycled materials are being explored for additive manufacturing applications, further enhancing its sustainability credentials.
Overall, the progress in additive manufacturing has been transformative, revolutionizing the way products are designed, prototyped, and manufactured across industries. With ongoing research and development efforts, additive manufacturing is expected to continue driving innovation, enabling new applications, and reshaping the future of manufacturing in the years to come.
Design For Additive Manufacturing:
Design for additive manufacturing (DfAM) is an approach to product design that takes into consideration the unique capabilities and constraints of additive manufacturing processes. Unlike traditional manufacturing methods, which often impose limitations on design due to constraints such as tooling, machining, and assembly requirements, additive manufacturing offers greater design freedom and flexibility. Here are key principles and considerations for designing for additive manufacturing:
1. Design Freedom:
Additive manufacturing enables the creation of highly complex geometries and intricate designs that may be difficult or impossible to produce using traditional methods. Designers have the freedom to create organic shapes, lattice structures, and lightweight components without the constraints imposed by subtractive processes.
2. Optimization for Additive Processes:
DfAM involves optimizing designs specifically for additive manufacturing processes, taking into account factors such as layer-by-layer deposition, support structures, and build orientation. Designers can leverage software tools and simulation techniques to optimize part orientation, minimize material usage, and reduce build time while ensuring structural integrity and functional performance.
3. Integration of Features:
Additive manufacturing allows for the integration of multiple features and functionalities within a single part, eliminating the need for assembly of separate components. Designers can consolidate complex assemblies into single-piece designs, reducing part count, assembly time, and potential points of failure.
4. Design for Performance:
DfAM enables the creation of custom-designed parts with optimized performance characteristics tailored to specific applications. By varying material properties, infill patterns, and internal structures, designers can enhance mechanical properties such as strength, stiffness, and fatigue resistance, as well as thermal and electrical conductivity.
5. Generative Design and Topology Optimization:
Generative design algorithms and topology optimization techniques can be employed to automatically generate optimized designs based on specified performance criteria and manufacturing constraints. These tools enable designers to explore a wide range of design alternatives and identify novel solutions that maximize performance while minimizing material usage and manufacturing complexity.
6. Design for Post-Processing:
DfAM involves considering post-processing requirements such as surface finishing, heat treatment, and support removal. Designers should design parts with appropriate tolerances, surface orientations, and support structures to facilitate post-processing operations and achieve desired surface quality and dimensional accuracy.
7. Material Selection:
DfAM requires careful consideration of material properties, compatibility with additive processes, and suitability for specific applications. Designers should select materials that offer the required mechanical, thermal, and chemical properties while considering factors such as cost, availability, and recyclability.
8. Design for Sustainability:
DfAM presents opportunities for designing products with sustainability in mind, such as optimizing material usage, reducing waste, and enabling on-demand production and localized manufacturing. Designers can explore bio-based, recycled, and recyclable materials, as well as lightweight designs that minimize energy consumption and environmental impact throughout the product lifecycle.
In conclusion, design for additive manufacturing (DfAM) is a holistic approach to product design that leverages the capabilities of additive manufacturing processes to create innovative, optimized, and sustainable products. By embracing DfAM principles and considering the unique opportunities afforded by additive manufacturing, designers can unlock new possibilities for product innovation, performance optimization, and manufacturing efficiency.
Additive Manufacturing Examples:
Additive manufacturing, also known as 3D printing, has revolutionized various industries by enabling the production of complex parts and prototypes with unprecedented design freedom and efficiency. Here are some examples of additive manufacturing applications across different sectors:
1. Aerospace:
- Aircraft components: Additive manufacturing is used to produce lightweight, complex aerospace components such as turbine blades, engine parts, brackets, and ducting systems. These parts can be optimized for performance, reducing weight and improving fuel efficiency.
- Prototyping: Aerospace companies utilize additive manufacturing for rapid prototyping of aircraft designs, allowing engineers to quickly iterate and test new concepts before full-scale production.
2. Healthcare:
- Medical implants: Additive manufacturing enables the production of custom-made medical implants, such as hip and knee replacements, cranial implants, and dental implants. These implants can be tailored to the patient’s anatomy for a better fit and improved patient outcomes.
- Prosthetics: Additive manufacturing is used to produce prosthetic limbs and orthotic devices, allowing for customization based on individual patient needs and preferences. 3D scanning technology can capture precise measurements for a perfect fit.
3. Automotive:
- Prototyping and tooling: Automotive manufacturers use additive manufacturing for rapid prototyping of vehicle components and tooling, reducing lead times and costs associated with traditional manufacturing methods.
- Spare parts: Additive manufacturing enables on-demand production of spare parts for older vehicles, eliminating the need for maintaining large inventories and reducing supply chain logistics.
4. Consumer Goods:
- Customized products: Additive manufacturing allows for the customization of consumer goods such as eyewear, footwear, jewelry, and accessories. Customers can personalize products according to their preferences, leading to greater customer satisfaction and brand loyalty.
- Home goods: Additive manufacturing is used to produce unique home goods and decor items, including furniture, lighting fixtures, and decorative objects. Designers can experiment with novel shapes and materials, creating one-of-a-kind pieces.
5. Engineering and Prototyping:
- Functional prototypes: Additive manufacturing facilitates the production of functional prototypes for testing and validation purposes in engineering and product development. Engineers can quickly iterate designs and make design changes without the need for expensive tooling.
- Jigs and fixtures: Additive manufacturing is used to produce custom jigs, fixtures, and tooling for manufacturing processes, improving efficiency and accuracy in assembly and production operations.
6. Education:
- Learning aids: Additive manufacturing is utilized in educational settings to create visual aids, models, and prototypes for teaching and learning purposes. Students can gain hands-on experience with 3D printing technology and learn about design principles and manufacturing processes.
These examples demonstrate the versatility and potential of additive manufacturing across various industries, from aerospace and healthcare to automotive, consumer goods, engineering, and education. As the technology continues to advance, the range of applications for additive manufacturing is expected to expand further, driving innovation and transforming manufacturing processes worldwide.
Advantage Of Additive Manufacturing?
Additive manufacturing, also known as 3D printing, offers numerous advantages over traditional manufacturing methods. Some of the key advantages include:
1. Design Freedom:
Additive manufacturing allows for the creation of highly complex geometries and intricate designs that are difficult or impossible to produce using traditional methods. Designers have the freedom to create organic shapes, lattice structures, and lightweight components without the constraints imposed by subtractive processes.
2. Rapid Prototyping:
Additive manufacturing enables rapid prototyping of parts and products, reducing lead times and costs associated with traditional prototyping methods. Design iterations can be quickly produced and tested, accelerating the product development cycle and improving time-to-market.
3. Customization:
Additive manufacturing enables the customization of products based on individual customer needs and preferences. Each part can be easily tailored to specific requirements without incurring additional tooling costs, allowing for mass customization and personalized manufacturing.
4. Reduced Material Waste:
Additive manufacturing is an inherently efficient process that minimizes material waste by only using the amount of material required to build the part. Unlike subtractive manufacturing methods, which produce significant amounts of waste material, additive manufacturing adds material layer by layer, resulting in minimal material wastage.
5. Lightweighting:
Additive manufacturing allows for the creation of lightweight components with optimized geometries and internal structures. By reducing material usage without sacrificing strength or performance, additive manufacturing enables the production of lightweight parts that are ideal for applications where weight is a critical factor, such as aerospace and automotive industries.
6. On-Demand Production:
Additive manufacturing enables on-demand production of parts and products, eliminating the need for large inventories and reducing supply chain logistics. Parts can be produced as needed, reducing inventory costs, lead times, and storage requirements.
7. Complex Assemblies Consolidation:
Additive manufacturing allows for the consolidation of complex assemblies into single-piece designs, reducing part count, assembly time, and potential points of failure. Components that traditionally required multiple parts to be manufactured separately and then assembled can now be produced as one integrated part.
8. Accessibility:
Additive manufacturing technology has become increasingly accessible and affordable, with desktop 3D printers available for use in homes, schools, and small businesses. This accessibility democratizes manufacturing, allowing individuals and organizations of all sizes to benefit from the advantages of additive manufacturing.
Overall, additive manufacturing offers a wide range of advantages, including design freedom, rapid prototyping, customization, reduced material waste, lightweighting, on-demand production, complex assemblies consolidation, and accessibility. These advantages have led to widespread adoption of additive manufacturing across various industries and are driving innovation and transformation in manufacturing processes worldwide.
Related Posts:
Additive manufacturing, explained
Digital Transformation Strategy for 2024
Supply Chain Optimization: Cost and Efficiency Mastery
Conclusion:
In conclusion, additive manufacturing stands at the forefront of innovation, offering unparalleled possibilities in product design, prototyping, and production. From its definition as a layer-by-layer fabrication process to the exploration of its diverse types and real-world examples across industries, this guide has provided a comprehensive understanding of additive manufacturing’s capabilities and applications. With advantages such as design freedom, rapid prototyping, and on-demand production, additive manufacturing continues to revolutionize traditional manufacturing methods, driving efficiency, customization, and sustainability. As the technology advances and new materials and processes emerge, the potential for additive manufacturing to transform industries and reshape the future of manufacturing is limitless.
Embracing additive manufacturing’s principles and harnessing its capabilities will undoubtedly lead to further innovation, unlocking new opportunities and driving progress in the ever evolving landscape of manufacturing.
FAQs:
What is the difference between additive manufacturing and traditional manufacturing methods?
Additive manufacturing, also known as 3D printing, builds objects layer by layer from digital models, while traditional manufacturing methods typically involve subtracting material from a solid block or shaping material through molding or casting processes. Additive manufacturing offers greater design freedom, rapid prototyping capabilities, and reduced material waste compared to traditional methods.
What are the main advantages of additive manufacturing?
Additive manufacturing offers several advantages, including design freedom to create complex geometries, rapid prototyping for quick iterations, customization for personalized products, reduced material waste through efficient use of materials, lightweighting for improved performance, on-demand production to eliminate large inventories, and complex assemblies consolidation for simplified designs.
What are the limitations of additive manufacturing?
While additive manufacturing offers numerous benefits, it also has limitations such as limited material selection compared to traditional methods, slower production speeds for large-scale production, surface finish and resolution constraints depending on the printing technology used, post-processing requirements for some parts, and challenges with certain material properties such as strength and durability.
What industries are using additive manufacturing?
Additive manufacturing is utilized across various industries, including aerospace for producing lightweight aircraft components, automotive for rapid prototyping and spare parts production, healthcare for customized medical implants and prosthetics, consumer goods for personalized products, engineering for functional prototypes and tooling, and education for teaching and learning purposes.
What is the future outlook for additive manufacturing?
The future of additive manufacturing looks promising, with ongoing advancements in materials, processes, and applications. Key developments include multi-material printing, improved process monitoring and control, integration of artificial intelligence and machine learning, adoption of sustainable materials and processes, scalability for mass production, and expansion into new industries and applications. Additive manufacturing is poised to continue driving innovation and reshaping the manufacturing landscape in the years to come.
All Images ‘Designed by Freepik‘
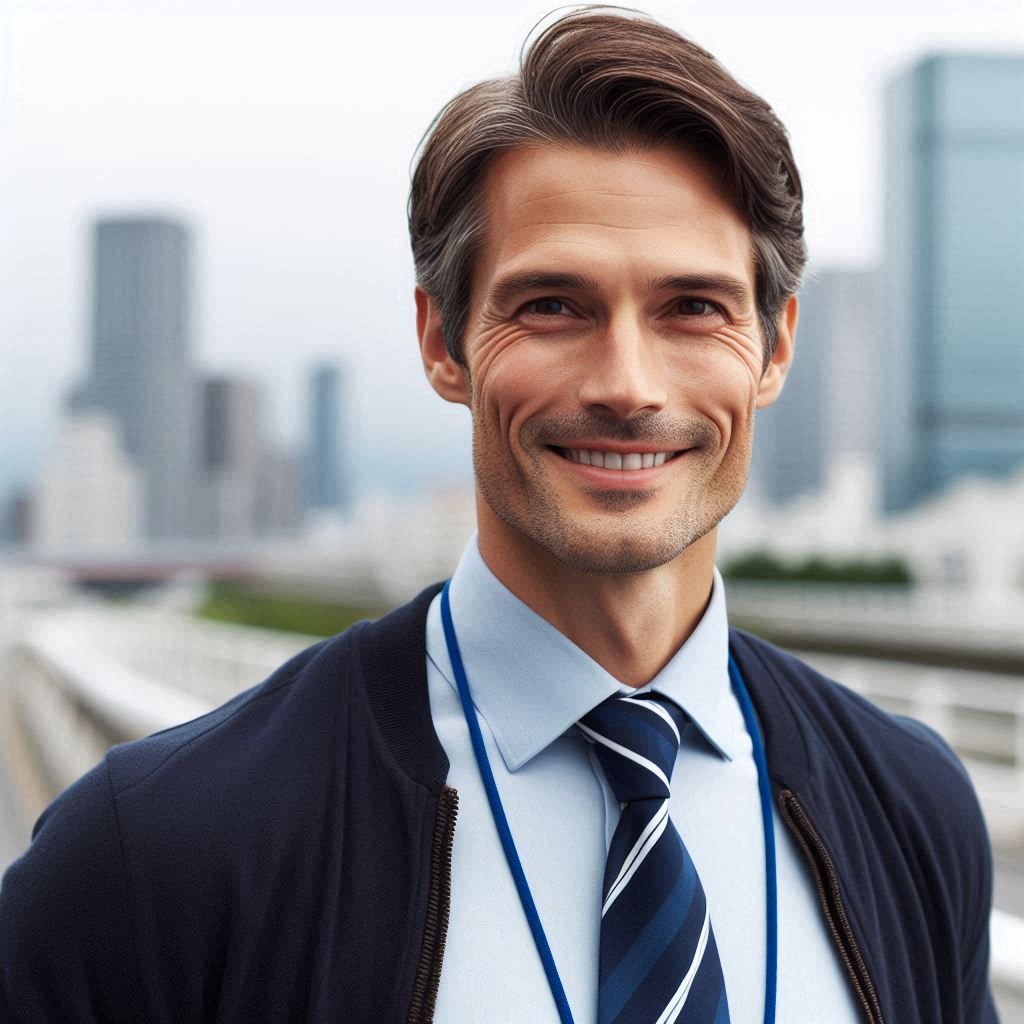
HA Chaudhary is an experienced engineer with 15 years in the mechanical and industrial sectors. Holding advanced degrees and multiple certifications in engineering, he combines technical expertise with a passion for writing to provide valuable insights into engineering innovations and business strategies. His content empowers professionals to excel in their fields and stay updated with the latest industry trends.
Wow, this was an amazing read! Your ability to articulate your thoughts so clearly and provide valuable insights is truly commendable. Keep up the fantastic work!
This post was incredibly helpful and inspiring. Your unique perspective and thoughtful analysis have given me a lot to think about. Looking forward to more of your amazing content!